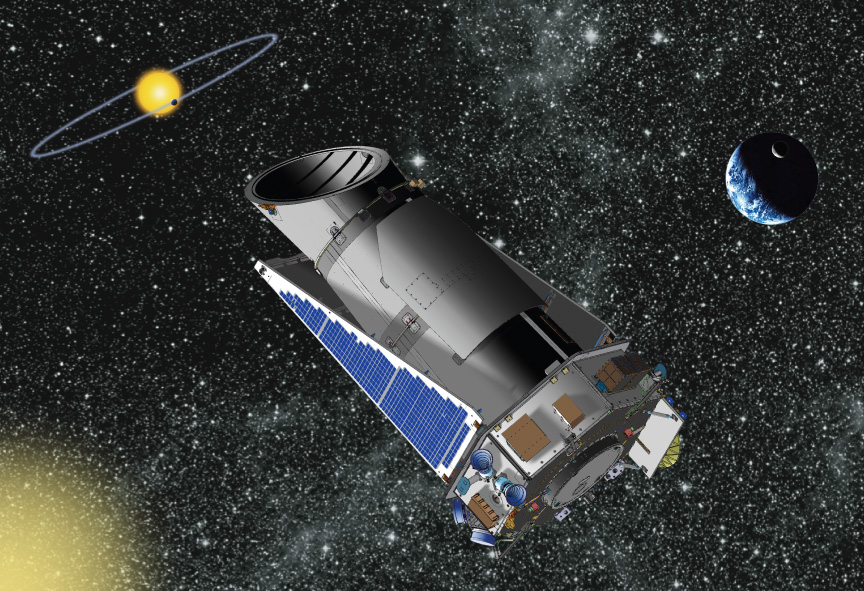
The Disequilibrium Fingerprint of Life: A Reflection on the Search for Exoplanets
The relentless human quest to discover extraterrestrial life has taken a major leap forward with a recent study published in Nature [1]. This article, titled “Disequilibrium Signatures as Biosignatures for Earth-Like Exoplanets,” delves into the exciting possibility of utilizing chemical disequilibrium as a beacon in the search for life on distant worlds. Traditionally, the hunt for exoplanets has focused on identifying planets within the habitable zone of their stars, where liquid water, a prerequisite for life as we know it, could exist. However, this approach has limitations, as it relies on the assumption that life as we understand it universally requires liquid water.
The present study proposes a more agnostic approach by exploring the concept of chemical disequilibrium as a potential biosignature. Chemical disequilibrium refers to a state where a system is not in its most thermodynamically stable state. On Earth, biological processes constantly push the atmosphere away from equilibrium. For instance, photosynthesis disrupts the atmospheric equilibrium between oxygen (O2) and methane (CH4), leading to a persistent disequilibrium. The authors propose that the detection of such a chemical disequilibrium signature on an exoplanet could be a strong indication of the presence of life.
The study’s brilliance lies in its focus on the quantification of this disequilibrium. The Gibbs free energy, a thermodynamic quantity, serves as a metric for quantifying the extent of disequilibrium within a system. The authors acknowledge that previous studies have explored the potential of disequilibrium biosignatures. However, a crucial gap existed – a lack of investigation into how observational uncertainties impact the ability to remotely sense a life-generated disequilibrium in a planetary atmosphere.
To address this gap, the researchers employed a two-pronged approach. First, they utilized an atmospheric retrieval tool, which is a computer program that simulates the path of light as it travels through a planetary atmosphere. This tool allows scientists to analyze the spectral signature of an exoplanet’s atmosphere, which can reveal the abundances of various gases present. Second, they coupled this retrieval tool with a sophisticated thermodynamic model. This model takes into account the complex interplay between atmospheric chemistry, pressure, and temperature to predict the expected equilibrium abundances of various gases in an exoplanet’s atmosphere.
By comparing the observed spectral signature with the model’s predictions of equilibrium abundances, researchers can identify the presence of any disequilibrium. The beauty of this approach lies in its independence from our current understanding of life’s prerequisites. Even if life on another planet utilizes completely different chemistries and energy sources, as long as it disrupts the equilibrium state of its environment, this method has the potential to detect it.
The study specifically focuses on the Proterozoic eon of Earth’s history, a period spanning from 2.5 billion to 541 million years ago. During this eon, the atmospheric abundances of certain disequilibrium pairs, such as oxygen (O2) and methane (CH4), are thought to have been particularly high. This makes Proterozoic Earth an ideal case study for demonstrating the detectability of such signatures using remote sensing techniques.
The findings of this study offer a powerful new tool for exoplanet astronomers. By incorporating the search for chemical disequilibrium into our battery of biosignature detection methods, we significantly broaden the scope of our search for extraterrestrial life. This approach holds immense promise for the future of space exploration, potentially enabling us to identify life on planets that may not necessarily harbor liquid water on their surfaces.
However, it is important to acknowledge the limitations and challenges associated with this method. One major hurdle is the complexity of atmospheric retrieval. Extracting reliable information about the composition of an exoplanet’s atmosphere from the faint starlight that filters through it is a demanding task. Additionally, the sensitivity of the method hinges on our understanding of the equilibrium states of various planetary atmospheres. If our models are incomplete or inaccurate, they could lead to misinterpretations of the observed disequilibrium signatures.
Furthermore, the possibility of false positives arising from non-biological processes that can induce chemical disequilibrium cannot be entirely ruled out. Geological phenomena, for instance, could potentially mimic the signatures of biological activity. Therefore, the detection of a single disequilibrium signature may not be conclusive evidence of life. A robust biosignature detection strategy would likely require the identification of multiple disequilibrium pairs that are not readily explained by non-biological processes.
In conclusion, the study presented in Nature marks a significant advancement in our quest to identify life beyond Earth. By introducing the concept of chemical disequilibrium as a potential biosignature, the authors offer a powerful and agnostic tool for exoplanet astronomers. While challenges and limitations remain, this novel approach has the potential to revolutionize our search for extraterrestrial life, opening doors to the exploration of a far wider range of planetary environments.
Reference article: https://www.nature.com/articles/s41550-023-02145-z